Prospects for the development of solar-hydrogen energy
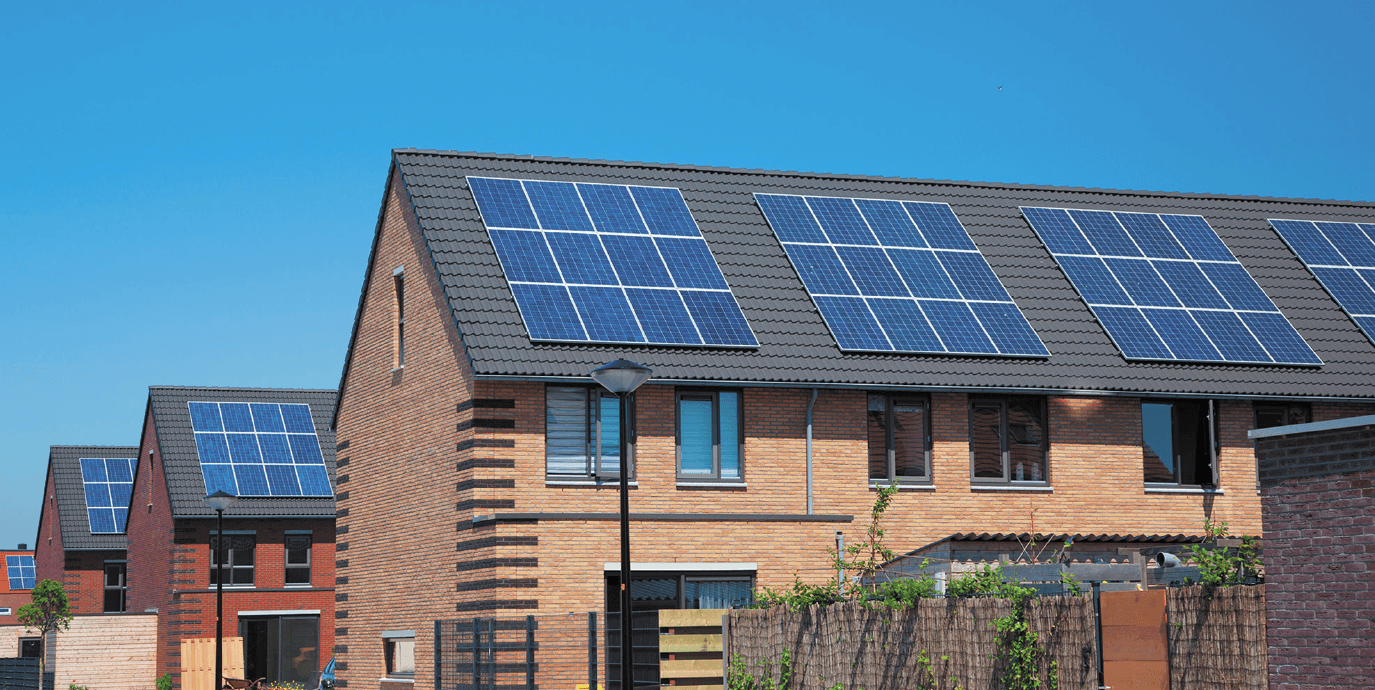
The traditional process of burning fossil fuels, which is the basis for obtaining thermal and electrical power, is one of the main causes of air pollution with substances harmful to humans and the environment. In addition, it causes an increase in the content of greenhouse gases in the atmosphere, leading to an increase in the global temperature of the planet. Thus, there is an inevitable change in the climate, the habitat of various animal species, forest boundaries, permafrost zones, etc. [1]. In 2018, climate change caused more than 300 natural disasters (93% are storms, forest fires, food shortages and droughts), which affected the lives of more than 68 million people and caused enormous economic damage in the amount of about $ 131.7 billion [2]. Another equally important problem is the limited reserves of traditional fuels and the constant growth of their consumption. Further depletion of fossil resources will gradually lead to an increase in their cost, which will ultimately cause new problems for the development of industry and society. Such challenges have caused great interest in renewable energy sources (RES), which can become a solution to the problems that have arisen. At the same time, in order to achieve carbon neutrality, the global share of RES, according to researchers' forecasts, should increase from 14 to 74% from 2018 to 2050.
When considering renewable energy as a whole, we can distinguish 5 main groups: solar energy, hydropower, wind energy, geothermal energy and the use of biofuels. At the same time, installations used on the basis of such primary energy have their own operational features, which are determined, among other things, by their location [3]. From a practical point of view, the use of solar energy alone can fully satisfy humanity's need for electrical and thermal energy, which makes this area of RES the most promising for research [4].
In the context of humanity, the Sun is a free and inexhaustible source of energy. Currently, this branch of renewable energy is developing rapidly (Fig. 1) [2].
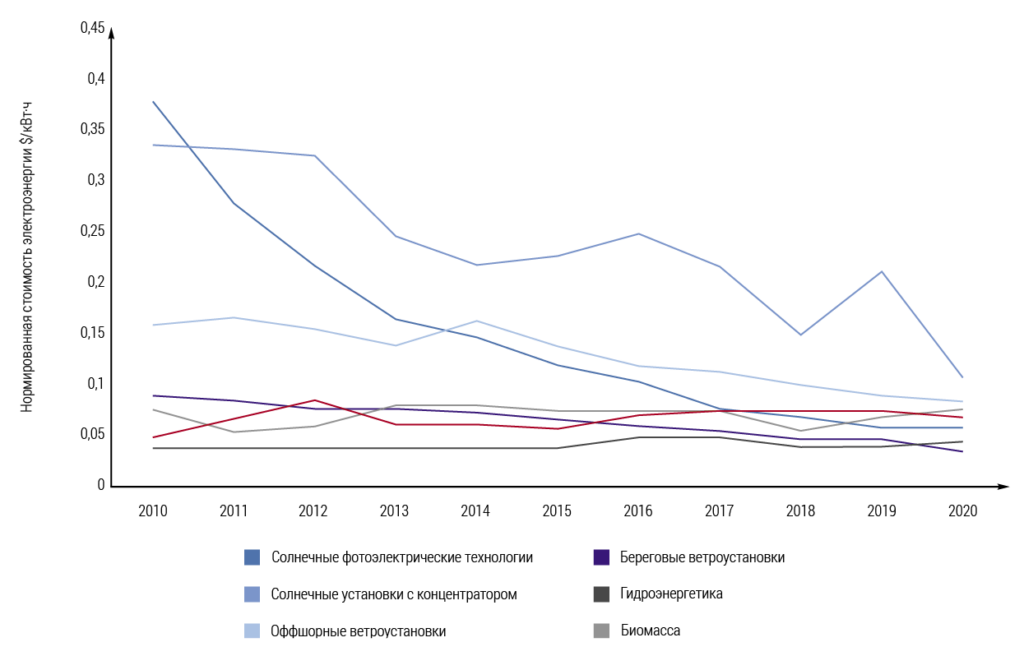
Taking into account only solar power engineering based on the use of photovoltaic converters, we note 2 main trends in the development of this technology: reducing the cost of solar cells (to achieve greater competitiveness) and increasing the efficiency of operation (to reduce the overall dimensions of the installations and the cost of the solar power system) [5]. Currently, the most common type of solar panels are silicon ones - they are the most widely represented on the modern market. However, the development of solar energy has led to the emergence of a large number of other types of solar cells, which are usually classified into three generations depending on the materials used in their manufacture. For a further description of the existing classification, please refer to the works [6-9]. The first generation includes monocrystalline silicon solar cells, which can be easily distinguished by their uniform color and shape (rectangular cells with beveled corners). Another type is polycrystalline panels, which consist of many individual crystals and have a rectangular appearance with unrounded edges. This generation also includes gallium arsenide panels, which are more efficient, but due to the presence of expensive, rare and toxic substances, they have not become widespread. However, the creation of silicon solar cells is a relatively expensive technology, so the search for cheaper methods of producing solar energy converters began.
The solution to this problem was second-generation converters, which include various types of thin-film solar cells. In turn, they are classified depending on the photovoltaic material that covers the substrate: cadmium telluride (CdTe), amorphous silicon (a-Si), copper indium diselenide (CIS), copper indium gallium selenium (CIGS) solar cells. These solar panels are cheaper to produce, have a flexible structure, and shading and elevated temperatures have less impact on their operation. However, their main drawback is relatively low efficiency, which prompted various developments of the third generation of solar cells.
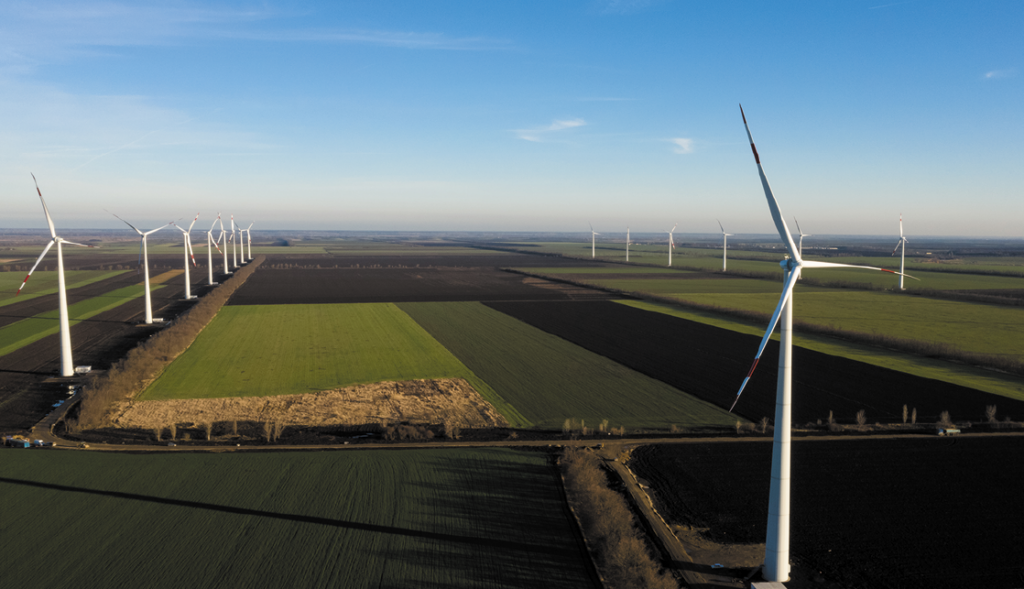
The third generation includes tandem solar panels and newer types of converters such as dye-sensitized solar cells; quantum dot solar cells; perovskite solar panels; organic solar panels; and copper zinc tin sulfide (CZTS) solar cells. The prospects for the development of this generation are associated with the development of a long-term technology that will be cost-effective for large-scale electricity production based on environmentally friendly materials with unlimited availability. Their many advantages can be justified by international investment and research aimed at increasing efficiency, reducing costs, and scaling up production on a large scale. Updated research by the US National Renewable Energy Laboratory allows us to trace the development of all the above-described generations of solar cells from 1975 to 2024 (Fig. 2) [8].
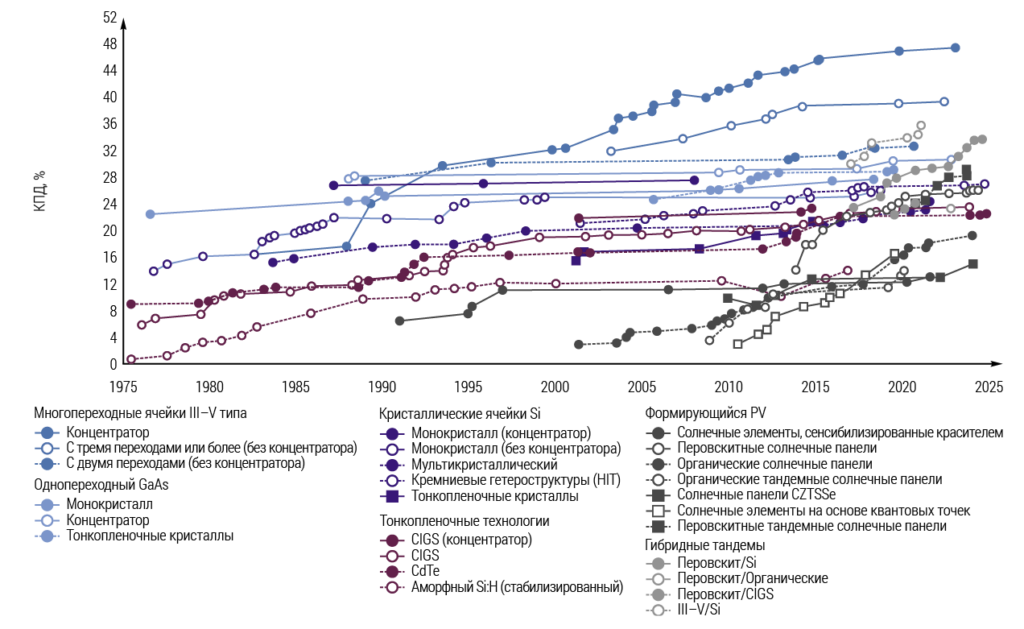
It follows from Fig. 2 that the efficiency of photovoltaic converters increases every year, while the area of the modules themselves decreases. Multijunction solar cells currently have the highest efficiency (more than 48%). It is also possible to note the rapid development of new types of third-generation photovoltaic converters (emerging PV). The integration of renewable energy sources based on such devices into the existing power system is associated with a number of problems that must be solved to ensure the stability, reliability and efficiency of its operation. The main problem is that the arrival of solar energy is inconsistent, due to which the power of the SES can fluctuate greatly due to weather conditions and other factors. Moreover, the more renewable energy sources are integrated into the network, the more difficult it is to ensure the stability of the power system.
A possible solution to the problem may be the use of energy storage systems and the implementation of capacity reservation algorithms based on them. In this area, hydrogen energy is especially worth noting, which has great potential for solving the problem.
Hydrogen is currently considered as a solution for decarbonization of various industries due to its advantages as an environmentally friendly fuel. When it is burned, there are no carbon dioxide emissions, which makes it an important tool for the development of a low-carbon economy. In addition, it has a high mass heat of combustion, which is almost 3 times higher than gasoline and is easily flammable [10]. Hydrogen, which was produced using renewable energy sources based on water electrolysis, is usually classified as "green" hydrogen. This technology helps to combine various sectors of the economy with renewable energy sources, and the general development of alternative energy and its production technologies gradually reduces the cost of such "green" hydrogen (Fig. 3) [11].
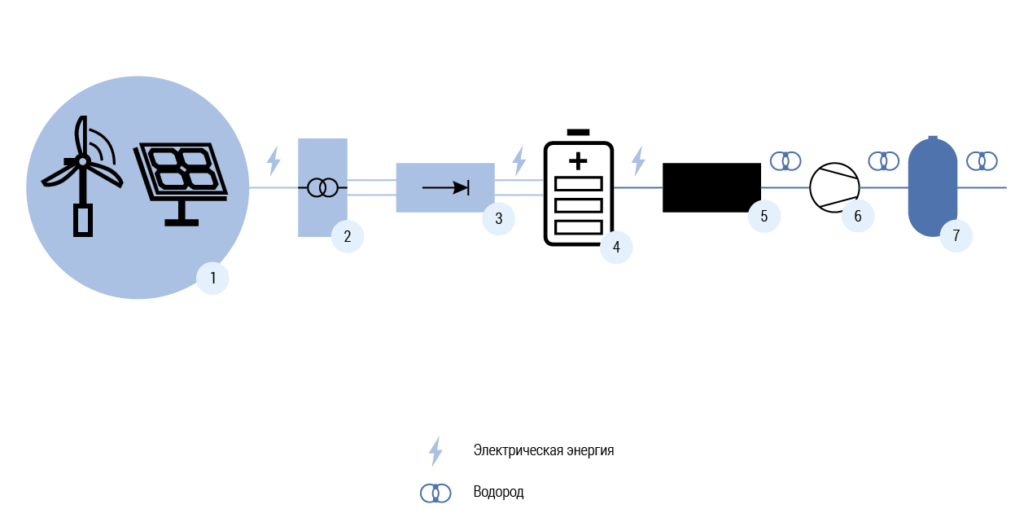
Despite the high cost of producing hydrogen as a fuel [12], the concept of combined solar-hydrogen energy is becoming increasingly popular. This system proposes to convert part of the incoming solar energy into hydrogen production, while it is possible to use energy obtained from both solar energy and accumulated hydrogen as fuel. The advantage of this method is the ability to intermediately accumulate solar energy to cover the load during non-sunny hours using the Power-to-gas (P2G) technology [13]. P2G technology uses the conversion of electrical energy into chemically bound energy in the form of a gaseous substance (usually hydrogen or methane), which, unlike electrical energy, is convenient for storage and further use. At the same time, this technology allows for a reduction in the need for natural gas for various objects of its consumption. It is worth noting that methane can also be obtained from accumulated hydrogen in accordance with the Sabatier reaction (formula 1) [14].
4H2 + CO2 = CH4 + 2H2O
In the context of the development of the designated solar-hydrogen energy concept, some successful projects implemented recently can be highlighted. These include: FH2R (Fig. 4, a) – the largest hydrogen production plant in Japan (it has a hydrogen production unit with a capacity of 10 MW, solar panels are used for generation) and the Sinopec plant in China (Fig. 4, b), which is capable of generating 600 million kWh per year (uses solar power plants) [15].
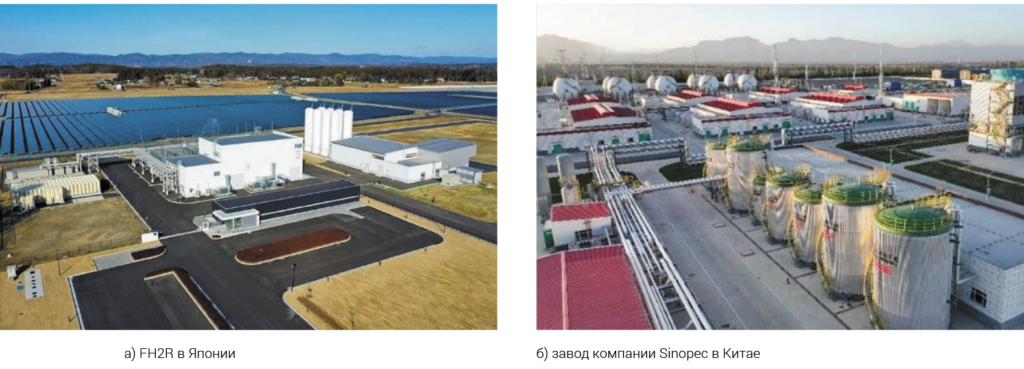
In Russia, research in this area is also currently underway, but with a slight shift in the focus of using primary energy for hydrogen production. According to information published on April 17, 2024 on the website of the Central Dispatch Office of the Fuel and Energy Complex, the Russian Federation intends to occupy 20% of the world hydrogen market by 2030. At the same time, it is reported that the main prerequisites for the development of hydrogen energy will be decarbonization and the transition to carbon-free sources. In this context, the Ministry of Energy of Russia speaks of the need to develop domestic technologies in the field of hydrogen energy, as well as to ensure the competitiveness of the country's economy in the context of the global energy transition. It is noted that the production of "green" hydrogen using renewable energy is planned in the Kaliningrad Region based on the conversion of wind energy. It is worth paying attention to the statement of LUKOIL, which plans to produce "green" hydrogen in the Krasnodar Territory in the amount of up to 13 tons per year. At the same time, it is not reported what type of energy will be used for this purpose [16]. Considering that solar energy in the structure of the energy balance of renewable energy sources for our country is not as developed as, for example, hydro or wind energy, it is difficult to name the existing large-scale production of "green" hydrogen based on solar energy, although there is significant technical potential for this, similar to the previously considered foreign projects. This fact actualizes the topic of the issue under consideration about the advisability of reserving the capacity of photovoltaic stations in the conditions of production of "green" hydrogen.
A typical grid-connected solar-hydrogen system (Figure 5) consists of a solar PV system, a water electrolyzer, a hydrogen gas storage tank, and a fuel cell. The outputs of the PV system and the fuel cell are connected to converters to convert the electrical current into the form required by the load. Excess PV energy is fed to the electrolyzer to generate “green” hydrogen, which is then stored in a dedicated pressurized tank for backup use. The stored hydrogen gas is then converted into electrical energy by the fuel cell to meet load needs during hours when PV generation is not available. To maintain the energy balance between generation and demand, the utility grid is only allowed to connect to cover any remaining loads not met by renewable energy production from the PV and fuel cells. Thus, the proposed combined configuration can ensure energy consumption security and power system operation reliability while increasing the supply volume of green energy and reducing the grid electricity procurement requirements [17].
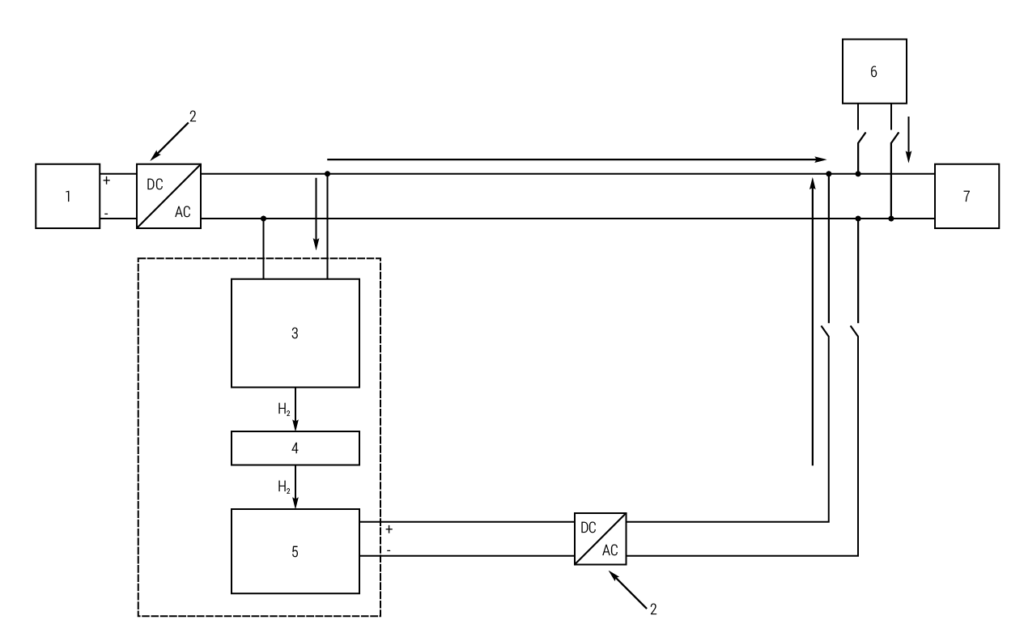
An example of an autonomous combined solar-hydrogen system is shown in Fig. 6. During the daytime, the available power sources for the house are solar energy from a photovoltaic solar panels array and energy from a fuel cell stack, which consumes hydrogen from a hydrogen tank during operation. Hydrogen is produced using an electrolyser when solar energy is available. After sunset, in this case, the fuel cell stack remains the only available power source in such a hybrid system [18].
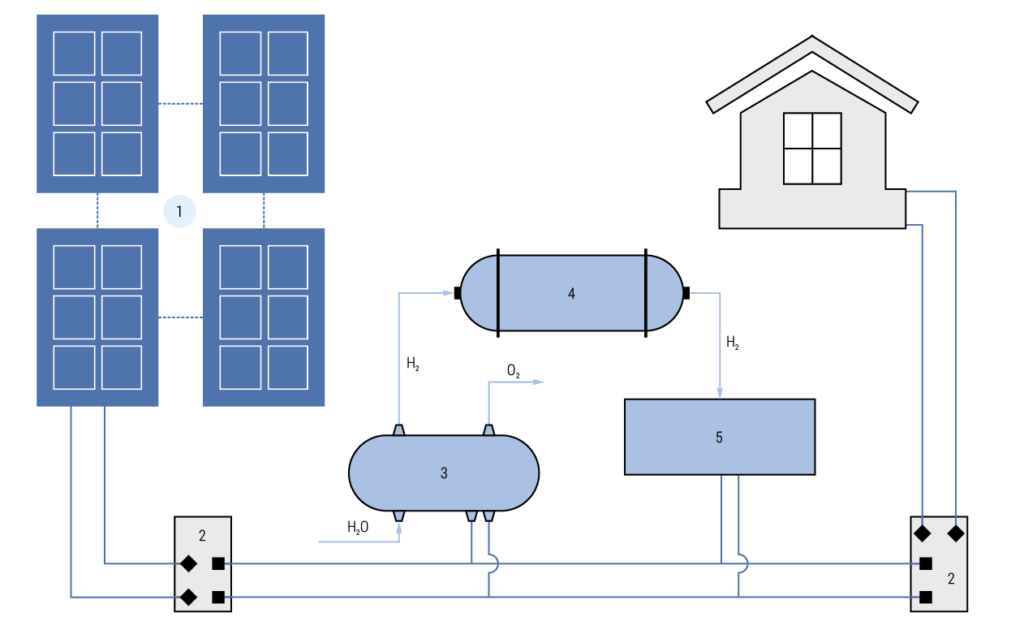
4 – hydrogen storage tank, 5 – fuel cell system
A successful project implemented in this direction already provides 100% autonomous energy supply for a residential building [19]. The entire roof of the building is covered with photovoltaic panels with an installed electrical capacity of 20 kW and an annual output of about 20 kW h. The electrical energy stored in the summer in the form of hydrogen is consumed in the winter by fuel cells. Hydrogen is stored in special tanks under a pressure of 32 bar. As a result, such a system made it possible to ensure complete autonomy of the residential building.
Currently, there is an increased interest in renewable energy sources, which is explained by the desire to decarbonize the production of energy capacities and reduce the share of hydrocarbon resources consumption. Analysis of numerous information sources has shown that among the variety of renewable sources, solar energy has a high rate of development, and the cost of producing electricity using photovoltaic converters is annually reduced and gradually approaches hydropower. Such successes are largely explained by the technical optimization of photovoltaic technologies, which have made it possible to significantly increase the efficiency of photovoltaic converters to almost 49%.
In the context of resolving the issue of reserving the electric capacity of photovoltaic stations and reorienting to the increasingly popular hydrogen energy, the development strategy of which has been approved by many countries in the modern world, the use of hydrogen as a method of energy storage is acquiring great practical significance. Analysis of pilot projects of this nature allows us to assert that the idea of hydrogen reserving has not only a theoretical basis, but also practical application. The main restraining factor so far is the cost of implementing such solutions.
energypolicy